Welcome, Guest |
You have to register before you can post on our site.
|
Forum Statistics |
» Members: 474
» Latest member: mega
» Forum threads: 424
» Forum posts: 973
Full Statistics
|
Online Users |
There are currently 20 online users. » 0 Member(s) | 19 Guest(s) Applebot
|
Latest Threads |
Don Smith Reactive Method
Forum: Alternative & Free Energy
Last Post: Mister.E.M.F.
05-31-2025, 05:02 PM
» Replies: 39
» Views: 5,822
|
Fixing the ‘Fatally Flawe...
Forum: General Talk
Last Post: Mister.E.M.F.
05-31-2025, 12:31 PM
» Replies: 1
» Views: 150
|
Hello
Forum: Announcements
Last Post: JoeLag
05-11-2025, 05:55 PM
» Replies: 0
» Views: 219
|
Ebner field
Forum: Alternative & Free Energy
Last Post: ephemeralt8
03-10-2025, 10:41 AM
» Replies: 0
» Views: 374
|
Kryptos Passage 4 Decoded
Forum: General Talk
Last Post: JoeLag
03-09-2025, 01:23 PM
» Replies: 0
» Views: 270
|
full pdf about TPU and RO...
Forum: Files
Last Post: Labidus
03-09-2025, 06:03 AM
» Replies: 2
» Views: 1,101
|
Instrumental Transcommuni...
Forum: General Talk
Last Post: ephemeralt8
03-08-2025, 12:12 AM
» Replies: 0
» Views: 214
|
How can you Accelerate li...
Forum: Alternative & Free Energy
Last Post: ephemeralt8
03-07-2025, 11:28 PM
» Replies: 0
» Views: 295
|
Richard Vialle Pdf files
Forum: Files
Last Post: Kangsteri
03-06-2025, 09:00 AM
» Replies: 0
» Views: 259
|
Neurophone / more informa...
Forum: General Talk
Last Post: Blehblah
02-16-2025, 02:54 AM
» Replies: 13
» Views: 12,424
|
|
|
Electrostatic Power Generator |
Posted by: JoeLag - 08-17-2024, 12:28 AM - Forum: Research And Concepts
- Replies (1)
|
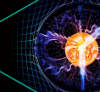 |
Overview:
This device appears to be an electrostatic power generator that leverages the principles of ionization, capacitance, and electrostatic interactions to generate and store electrical energy. The setup involves a combination of hybrid ion valves functioning as capacitors (similar to Leyden jars), copper coils for high-frequency (HF) filtering, and a configuration of dissimilar metals to create a potential difference and generate current.
Components Breakdown:
- Hybrid Ion Valves as Capacitors (Leyden Jar Configuration):
- These are used to store charge and create a potential difference. The ion valves function similarly to Leyden jars, where a dielectric material (ionized air in this case) is sandwiched between conductive plates or surfaces (MG mesh electrodes).
- The center rod inside each ion valve is a copper coil. This coil serves two purposes: filtering high-frequency signals and helping ionize the air around it.
- Copper Coil for HF Filtering and Ionization:
- The copper coil in the center of the ion valve serves as a high-frequency filter, ensuring that only the desired frequencies are allowed through while unwanted frequencies are filtered out.
- Additionally, the high voltage applied to this coil creates an intense electric field around it, which ionizes the surrounding air. This ionized air acts as a dielectric medium with enhanced properties, increasing the capacitance of the system.
- Dissimilar Metals Reaction:
- The device utilizes dissimilar metals (e.g., magnesium mesh and other metallic components) to create a galvanic reaction. This reaction contributes to generating a real potential difference (voltage) and current within the magnetic field of the system.
- This galvanic effect works alongside the electrostatic storage and helps to maintain a steady potential difference, further charging the internal capacitors.
- MG Mesh Electrodes and Ionized Air Dielectric:
- The internal capacitors are made of magnesium (MG) mesh electrodes with a small gap of air between them. This air is ionized by the high voltage field generated by the copper coil, which significantly enhances the dielectric properties of the air.
- As the dielectric constant of the air increases due to ionization, the capacitance of these internal capacitors increases, allowing them to store more energy.
- Cap Dump Outputs:
- The energy stored in the capacitors is periodically released or "dumped" into the circuit, providing a burst of electrical energy. This is the "cap dump" output mentioned in the diagram.
- The enhanced capacitance due to ionized air allows for more substantial energy storage and, consequently, more powerful outputs when the stored energy is released.
How It All Comes Together:- The device begins by generating a high voltage through an electrostatic generator (depicted by the hand-crank mechanism on the left).
- This high voltage is applied to the hybrid ion valves, which store the energy in the form of an electrostatic charge.
- The copper coils inside these valves help filter out unwanted frequencies and ionize the air around the MG mesh electrodes.
- The dissimilar metals create a small but constant potential difference, contributing to the overall energy generation process.
- The internal capacitors, with their enhanced capacitance due to the ionized air, store a significant amount of energy, which is then periodically released to produce a high-power output.
This system combines traditional electrostatic principles with innovative uses of ionization and materials science to create a power-generating and storing device that capitalizes on high voltage and high-frequency effects. The enhanced dielectric properties due to ionized air and the galvanic reactions of dissimilar metals make this setup potentially more efficient than conventional electrostatic generators.
|
|
|
The Magnetic Rectifier |
Posted by: JoeLag - 08-16-2024, 06:41 PM - Forum: Research And Concepts
- Replies (2)
|
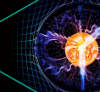 |
Understanding the Magnetic Rectifier: Analyzing a Novel AC to DC Conversion Technique
The concept illustrated in the provided image describes a magnetic rectifier designed to convert alternating current (AC) to direct current (DC) using the magnetic properties of a core and coils, combined with the influence of permanent magnets. This method is distinct from conventional semiconductor-based rectifiers, offering a different approach to rectification that could be of interest in various energy conversion and harvesting applications.
How the Magnetic Rectifier Works
- Coils and Cores:
- The rectifier consists of two cores, each with a coil wound around it. The coils are wound in the same direction using No. 30 S.S.C. wire (which likely stands for single-stranded copper wire). Each core has 1,000 feet of wire wound onto it.
- The cores are cylindrical, measuring 2 inches long by ⅞ inches in diameter, and are likely made of soft iron to enhance magnetic flux concentration.
- Permanent Magnets:
- Two bar magnets are positioned as close as possible to the cores without touching them. These magnets are pivotal in the operation of the rectifier. The diagram specifies that the like poles of these magnets should face each other, creating a strong magnetic field across the gap between them.
- AC Input and Grounding:
- The AC line is connected such that one side is grounded to one core, and the other side of the AC line is connected to the second core. This setup allows the alternating current to flow through the coils wound around the cores.
- Rectification Process:
- The magnetic field created by the permanent magnets interacts with the AC current flowing through the coils. As the AC current oscillates, the changing magnetic field in the cores due to the interaction with the permanent magnets forces the current to flow in a single direction when taken from the contact points at the pivot of the magnets. This results in a rectified DC output.
- The output DC is taken from the contact points holding the permanent magnets. The magnetic field from the bar magnets induces a directional flow of current, effectively rectifying the AC input into DC output.
Key Principles at Play
- Magnetic Saturation and Switching:
- The operation of this rectifier hinges on magnetic saturation and switching effects caused by the alternating magnetic field. As the AC current oscillates, it alternates the magnetization of the cores, which interacts with the permanent magnetic field to favor current flow in one direction more than the other.
- Use of Soft Iron Cores:
- Soft iron cores are used because they can easily be magnetized and demagnetized, which is essential for the switching action that occurs with each cycle of AC input.
- Symmetrical Magnetic Field:
- The like poles of the permanent magnets facing each other create a symmetrical and opposing magnetic field across the cores. This configuration might help in maintaining a more stable and steady DC output by ensuring that the magnetic influence is consistent as the AC current changes direction.
Application in Modern Research and Energy Systems
- Energy Harvesting:
- This magnetic rectifier could be adapted for low-power energy harvesting applications, where ambient AC electromagnetic fields are rectified into usable DC. Its simplicity and lack of semiconductor components make it potentially useful in environments with high electromagnetic noise or where conventional diodes might fail due to thermal or electrical stresses.
- Passive Rectification:
- In scenarios where passive components are preferred over active components (e.g., in high-radiation or high-temperature environments), this rectifier could provide a reliable means of converting AC to DC without the need for traditional semiconductors.
- Electromagnetic Compatibility:
- Given its reliance on magnetic fields rather than direct electrical connections to rectify current, this approach might offer unique benefits in systems where electromagnetic compatibility (EMC) is a concern. It could be used to design rectifiers that minimize electrical noise or interference.
- Exploring Negative Resistance:
- In line with your research into negative resistance and non-linear effects, this magnetic rectifier could be part of a broader exploration into non-linear magnetic systems. The magnetic interaction here introduces non-linearity that could be exploited in advanced energy systems or novel power conditioning technologies.
Conclusion
The magnetic rectifier described here presents a unique method of converting AC to DC using magnetic fields and soft iron cores influenced by permanent magnets. Its application could be particularly relevant in energy harvesting, passive rectification, or environments where conventional semiconductor rectifiers are less effective. By exploring this approach further, you could integrate it into modern systems where efficiency, simplicity, and durability are critical, potentially expanding the scope of your research into novel energy conversion technologies.
|
|
|
Gravity Wave Device |
Posted by: JoeLag - 08-11-2024, 07:48 PM - Forum: Research And Concepts
- Replies (3)
|
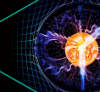 |
The diagram you've provided is labeled as a "G Wave Device" by Joel Lagace. It appears to be a conceptual design for a system that generates or manipulates gravitational waves or some form of thrust force through electromagnetic means. Let’s break down the components and speculate on how this device might operate.
Components and Configuration:
- Tank Capacitor:
- Function: The tank capacitor is likely part of a resonant circuit, possibly an LC circuit (inductor-capacitor circuit), that is designed to store and release energy in a controlled manner. The capacitor stores electrical energy and, in conjunction with the inductor (primary coil), oscillates at a specific frequency.
- Role in Resonance: This capacitor, paired with the primary coil, helps establish a resonant frequency for the circuit. The resonance would allow the system to build up large oscillating currents and magnetic fields with minimal input power.
- Primary Coil:
- Function: The primary coil, which is coupled with the tank capacitor, generates a magnetic field when current flows through it. This coil is a key part of the resonant circuit and is responsible for producing the magnetic field that interacts with other parts of the device.
- Electromagnetic Interaction: The coil’s magnetic field likely interacts with the magnetic field lines shown in the diagram, contributing to the generation of the G waves or thrust forces.
- Controller (400 Hz, Phased 180 Degrees):
- Purpose: This controller modulates the current flowing through the coils at a frequency of 400 Hz and ensures that the current in the coils is 180 degrees out of phase. This phasing is crucial for creating alternating magnetic fields that could interfere constructively or destructively, depending on the desired outcome.
- Frequency Modulation: The specific choice of 400 Hz might be related to the natural resonant frequency of the device or a frequency at which the device is most efficient at generating the desired effects.
- Coils (High Current Modulation):
- Function: These coils are modulated with high current, which likely means they are designed to handle large amounts of power. The modulation of these coils would create varying magnetic fields, which could interact with each other and the environment to produce a thrust force or gravitational wave effect.
- Magnetic Field Interaction: The diagram shows magnetic field lines around these coils, suggesting that they play a crucial role in shaping and directing the magnetic fields generated by the system.
- Magnetic Field Lines:
- Visualization: The diagram shows magnetic field lines (green arrows) emanating from the central circular structure. These lines represent the path along which the magnetic flux travels, and they are likely manipulated by the currents in the coils to produce the desired force.
- Interaction with Current: The yellow arrows represent the direction of current flow within the circular structure. The interaction between the current and the magnetic field lines could be responsible for generating the thrust force or the gravitational wave effect.
- Thrust Force:
- Generation: The thrust force is indicated at the top of the device, suggesting that the interaction of the magnetic fields and currents within the device produces a mechanical force. This could be due to the Lorentz force, where a current-carrying conductor in a magnetic field experiences a force.
- Possible Gravitational Wave Production: If this device is intended to generate gravitational waves, the thrust force might be a byproduct of the manipulation of spacetime, where the electromagnetic fields interact with the fabric of space to produce ripples or waves.
- Mass or Sensor:
- Role: This component at the top right could either be a mass that responds to the generated thrust force or a sensor that measures the effects of the device. If it’s a sensor, it might be detecting changes in gravitational fields or measuring the force produced by the device.
Speculative Working Principle:
- Electromagnetic Interaction:
- The device operates by generating and modulating strong magnetic fields through the coils and capacitors. The phased modulation at 400 Hz could create conditions where the magnetic fields interfere in a way that produces a net force or generates gravitational waves.
- Resonance and Energy Build-Up:
- The LC circuit formed by the tank capacitor and primary coil is likely tuned to resonate at a specific frequency, allowing the system to build up significant energy. This energy is then used to drive the high-current coils, creating intense magnetic fields.
- Thrust or Gravitational Wave Production:
- The interaction between the magnetic fields and the structure of the device could lead to the production of a thrust force. If the device is designed to manipulate gravitational waves, the alternating magnetic fields might induce perturbations in spacetime, generating the desired waves.
- Phased Magnetic Field Manipulation:
- The 180-degree phasing ensures that the magnetic fields produced by the coils are carefully timed to either enhance or cancel each other out in specific regions. This careful control of the magnetic fields is crucial for directing the force or wave generation in a controlled manner.
Conclusion and Further Exploration:
This G Wave Device by Joel Lagace appears to be an advanced concept aimed at generating either a thrust force or gravitational waves through the precise control and modulation of magnetic fields. The use of resonant circuits and phased magnetic fields suggests a deep understanding of electromagnetic principles, possibly coupled with speculative or emerging theories in physics.
Let's delve deeper into the theoretical foundations, potential applications, and challenges associated with the G Wave Device concept.
Theoretical Foundations:
- Electromagnetic Fields and Resonance:
- The device utilizes electromagnetic fields, which are fundamental to many alternative energy and propulsion concepts. The resonance created by the LC circuit (tank capacitor and primary coil) is key to amplifying the energy within the system. Resonance allows for the buildup of large oscillating currents and magnetic fields with minimal input power, which is crucial for achieving the desired effects.
- Lorentz Force:
- The Lorentz force is the force experienced by a charged particle moving through a magnetic field, given by the equation F=q(E+V x B)
- In this device, the current-carrying coils interact with the magnetic fields they generate, producing a force. If designed correctly, this force could be directed to produce thrust.
- Gravitational Waves and Spacetime Manipulation:
- Gravitational waves are ripples in spacetime caused by accelerated masses, as predicted by Einstein's General Theory of Relativity. While conventional gravitational waves are generated by astronomical events (like merging black holes), the idea here might be to use electromagnetic fields to induce similar effects on a much smaller scale. This would involve advanced theoretical physics, possibly leveraging concepts like electromagnetic stress-energy tensors to influence spacetime.
- Phased Magnetic Fields:
- The use of phased magnetic fields (with a 180-degree phase difference) is crucial for creating constructive or destructive interference patterns. This can either amplify the effects (constructive interference) or cancel out unwanted interactions (destructive interference). The careful control of these fields might allow for the generation of directed thrust or other exotic effects like gravitational wave emission.
Potential Applications:
- Propulsion Systems:
- If the device can generate a significant thrust force through electromagnetic means, it could be a candidate for advanced propulsion systems, particularly for space exploration. Unlike conventional propulsion that relies on expelling mass (rocketry), this system might offer a form of "reactionless" propulsion, reducing the need for fuel.
- Energy Generation:
- The device might be able to convert ambient or external energy sources into usable electrical power, possibly with very high efficiency if the resonant conditions are optimized. This could be applied in scenarios where conventional energy generation is impractical, such as deep-space missions or remote locations.
- Gravitational Wave Research:
- If the device indeed interacts with or generates gravitational waves, it could serve as a research tool in the field of gravitational wave detection and manipulation. This would be groundbreaking, as it could provide a new method to study spacetime and gravitational phenomena on a smaller, more controllable scale.
- Field Manipulation and Shielding:
- The ability to generate and control strong magnetic fields could have applications in shielding sensitive equipment from external electromagnetic interference or even in controlling the behavior of charged particles in a given space (such as in particle accelerators or plasma containment systems).
Challenges and Considerations:
- Technical Challenges:
- Material Science: The materials used must withstand high currents and strong magnetic fields without degrading or introducing unwanted losses. High-temperature superconductors could be a candidate material if cooling systems are feasible.
- Precision Control: The phasing and modulation of currents must be precisely controlled to maintain the desired electromagnetic field interactions. This requires advanced electronics and possibly real-time feedback systems to adjust the phase and amplitude dynamically.
- Theoretical Validation:
- Scientific Scrutiny: The underlying physics, especially if it claims to generate gravitational waves or "reactionless" thrust, would need to be rigorously tested and validated against existing physical laws. There may be skepticism, as such concepts challenge conventional physics, so careful experimentation and peer-reviewed research are essential.
- Resonance Stability: Maintaining resonance in a dynamic system can be challenging, especially under varying external conditions. The system must be designed to adapt or stabilize itself to avoid drifting out of resonance.
- Energy Efficiency:
- Power Input vs. Output: The system's overall energy efficiency needs to be carefully evaluated. If the energy required to maintain the system's operations exceeds the output or the benefits (e.g., thrust produced), it might not be practical.
- Heat Dissipation: High currents and magnetic fields can generate significant heat, which needs to be managed effectively to prevent damage or loss of efficiency.
- Ethical and Safety Considerations:
- Unintended Consequences: Generating strong magnetic fields or gravitational waves could have unintended effects on nearby electronics, biological tissues, or even the environment. Proper safety measures and thorough testing are crucial.
- Regulatory Approvals: Devices that manipulate fundamental forces might be subject to regulatory oversight, particularly if they involve high energy levels or could have far-reaching effects.
Conclusion:
The G Wave Device represents a fascinating blend of theoretical physics and advanced engineering, with potential applications in propulsion, energy generation, and fundamental research. However, it also presents significant challenges, both in terms of technical implementation and theoretical validation. Moving forward, detailed experimentation, careful design, and open scientific collaboration will be crucial to explore the full potential of this concept.
Creating a conceptual version of the G Wave Device using readily available materials and components involves making practical decisions about what can be sourced and implemented with existing technologies. Here’s how we can break down the process, focusing on materials, components, and known industrial brands for an approachable design:
1. Core Components and Materials:
Primary Coil and Secondary Coil:- Material: Use copper wire for the coils due to its excellent conductivity and availability.
- Specification:
- Wire Gauge: 14 to 18 AWG, depending on the current handling requirements.
- Insulation: Use enamel-coated magnet wire to ensure efficient winding with minimal losses.
- Example Part Number: Magnet Wire AWG 18 - Remington Industries
- Coil Form: You can use plastic or ceramic coil forms to wind the wire, which can be sourced from suppliers like McMaster-Carr or custom-made using 3D printing.
Tank Capacitor:- Material: High-quality, high-voltage capacitors are necessary for the tank circuit to handle the energy storage and release cycles.
- Specification:
- Capacitance: 10 µF to 100 µF (depending on the frequency of operation and desired resonance).
- Voltage Rating: 1000V or higher, depending on the expected voltage swings in the circuit.
- Example Part Number: Cornell Dubilier 940C30P1K-F - 10µF, 1000V Polypropylene Capacitor.
2. Control System:
Frequency Generator:- Purpose: To generate the necessary control signals at the specified frequency (400 Hz in the diagram) with 180-degree phase shifts.
- Specification:
- Frequency Range: 0.1 Hz to 1 MHz (to allow for tuning and experimentation).
- Phase Control: Ability to set phase shifts with fine precision.
- Output: Should be capable of driving the coils directly or through a power amplifier.
- Example Product: Agilent/Keysight 33500B Series Waveform Generator - Known for its precision and reliability.
- Alternative: Rigol DG1022Z Function Generator - A more budget-friendly option with similar functionality.
Power Amplifier:- Purpose: To amplify the signal from the frequency generator to drive high-current coils.
- Specification:
- Power Output: Depending on the coil's current requirements, an amplifier capable of delivering several amps at the desired voltage might be necessary.
- Example Product: Texas Instruments TPA3255EVM Evaluation Module - Capable of delivering high power output with efficient heat management.
3. Supporting Components:
High Current Modulation Coil:- Material: Similar to the primary and secondary coils, using thicker gauge copper wire if higher current is needed.
- Specification: 10 AWG to 14 AWG copper wire with appropriate insulation.
- Example Part Number: Southwire 10 AWG Copper Magnet Wire - Easily available in different gauges.
Magnetic Core (If Needed):- Material: Soft iron or ferrite cores are common for inductors to concentrate and enhance the magnetic field.
- Specification: Choose a core material that suits the frequency and power levels.
- Example Part Number: Micrometals Iron Powder Cores - Various cores available for different applications.
4. Assembly and Conceptual Build:
Step 1: Construct the LC Tank Circuit- Coil Winding: Wind the primary coil using the chosen wire gauge on a non-conductive form. Calculate the number of turns based on the desired inductance and the resonance frequency.
- Capacitor Connection: Connect the chosen tank capacitor in parallel with the primary coil to form the resonant circuit.
Step 2: Set Up the Frequency Generator and Amplifier- Frequency Generator Configuration: Program the generator to output a 400 Hz sine wave, ensuring the ability to adjust the phase. Connect the output to the power amplifier.
- Amplification: Use the amplifier to boost the signal from the frequency generator to a level sufficient to drive the coils.
Step 3: Connect and Test the Coils- High Current Modulation: Connect the output of the power amplifier to the high current modulation coil, ensuring it’s capable of handling the current without overheating.
- Secondary Coil Integration: The secondary coil should be placed in proximity to the primary coil, ensuring proper coupling for magnetic field interaction.
Step 4: Monitor and Measure- Mass or Sensor Integration: Attach a sensor or mass to the location where the thrust force is expected, and use instruments like a force gauge or accelerometer to measure any generated forces.
- Data Collection: Use an oscilloscope or data acquisition system to monitor the current, voltage, and magnetic field interactions, ensuring the system operates as expected.
5. Tuning and Optimization- Frequency Tuning: Adjust the frequency slightly around 400 Hz to find the optimal resonant point, which might differ slightly depending on the actual inductance and capacitance values.
- Phase Adjustment: Experiment with the phase settings to see how different phase shifts affect the magnetic field interactions and any generated thrust.
Conclusion:
This conceptual version of the G Wave Device uses well-known components and materials that are accessible in the industrial and hobbyist markets. By building and experimenting with this setup, you can explore the principles behind electromagnetic field manipulation and its potential applications in propulsion or energy generation.
|
|
|
Tesla's Radiant Energy |
Posted by: JoeLag - 08-11-2024, 07:19 PM - Forum: Research And Concepts
- Replies (2)
|
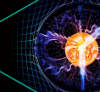 |
Let’s analyze the components and speculate on how this system might work.
Components and Configuration:
- Rectangular Element (Top-Left):
- Function: This element appears to represent a receiver or collector of some form of energy, possibly electromagnetic waves (like radio waves, light, or even some form of directed energy). The parallel lines indicate that this component is receiving or collecting incoming energy.
- Material: It could be made from a material designed to absorb or collect electromagnetic radiation efficiently, such as a specialized antenna, solar panel, or other energy-harvesting surface.
- Circuit Controller:
- Purpose: The circuit controller likely regulates the flow of electricity in the system. It might be designed to control when the collected energy is stored, transferred, or used to power a load.
- Operation: This could involve switching mechanisms, possibly using relays or solid-state switches, to modulate the current flow based on the energy collected and the requirements of the load.
- Transformer:
- Purpose: The transformer is crucial for adjusting the voltage and current levels of the electricity generated or collected. It might step up the voltage for efficient transmission or step it down for use in the load.
- Magnetic Interaction: Transformers work on the principle of electromagnetic induction, where a varying current in the primary winding induces a current in the secondary winding, typically with a change in voltage depending on the turns ratio of the coils.
- Load:
- Purpose: The load represents the device or system that consumes the electricity generated by this setup. This could be anything from a light bulb to a more complex system like a motor or battery charger.
- Electrical Characteristics: The nature of the load would dictate how the rest of the system needs to be designed. For instance, if it's a resistive load, the system must ensure that the voltage and current supplied match the load's requirements.
- Grounded Element:
- Purpose: The element at the bottom that is grounded might represent an energy source or a reference point for the circuit. It could be connected to an earth ground, acting as a return path for the electrical current or as a stabilizing element to maintain consistent potential in the circuit.
- Energy Source: Alternatively, this could represent a form of energy tapping, possibly from environmental sources like the Earth’s magnetic field, ground currents, or even tapping into some unconventional energy source.
Speculative Working Principle:
- Energy Collection:
- The incoming energy collected by the rectangular element could be any form of ambient or directed energy, such as solar radiation, radio frequency waves, or even mechanical vibrations converted to electrical signals.
- This energy is then processed and regulated by the circuit controller.
- Transformation and Regulation:
- The circuit controller ensures that the energy collected is within the appropriate parameters before it is sent to the transformer. This might involve rectifying an AC signal to DC, filtering, or controlling the flow to prevent overloads.
- The transformer adjusts the energy to a suitable voltage and current level for the load.
- Powering the Load:
- The load is powered by the energy that has been transformed and regulated. This could be a continuous process if the incoming energy is steady or intermittent if it relies on variable sources (e.g., solar power during the day).
Additional Speculative Enhancements:- Resonant Tuning: There could be a resonant circuit involved that is tuned to maximize the energy capture from specific frequencies of electromagnetic waves, enhancing efficiency.
- Energy Storage: Though not shown, there could be a storage element like a capacitor or battery in the system to store excess energy for use during times when the incoming energy is insufficient.
- Dynamic Load Balancing: The circuit controller might dynamically adjust the energy flow to the load based on real-time demands, ensuring optimal use of the collected energy.
Conclusion and Further Exploration:
This system likely aims to convert some form of ambient or directed energy into usable electrical power, regulated and transformed for a specific load. The grounded element might suggest a connection to environmental energy sources or just a stable reference point for the circuit.
|
|
|
Schematic Of The Kromrey Converter |
Posted by: JoeLag - 08-11-2024, 06:58 PM - Forum: Research And Concepts
- Replies (1)
|
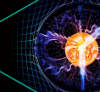 |
Let's break down the core components and the working principles as they are understood.
Components and Configuration:
- Non-Magnetic Shaft:
- The shaft in this device needs to be made of a non-magnetic material to avoid interference with the magnetic flux. This is crucial as it ensures the magnetic fields generated by the components are not disrupted, which could otherwise lead to inefficiencies or even failure in generating the desired effects.
- Magnets (N and S):
- The presence of labeled "N" (North) and "S" (South) indicates the use of permanent magnets. These magnets are likely arranged in a manner that their fields interact with coils or other components of the device to generate electrical effects.
- Coils (B1, B2):
- The labeled components "B1" and "B2" likely represent coils or windings. When magnets move relative to these coils, an electromotive force (EMF) is induced according to Faraday's Law of Induction. The interaction between the moving magnetic fields and the coils is central to the converter's operation.
- Springs/Clappers:
- There are mentions of springs or clappers, which might be used to modulate the movement or positioning of components, potentially to regulate the interaction between the magnetic fields and the coils.
- ALW (Aperture or Air Gap):
- This might represent an air gap or an aperture within the magnetic circuit. The control of the air gap is essential in determining the magnetic flux density in various parts of the circuit, affecting the output and efficiency of the converter.
Working Principle:
The Kromrey Converter is thought to operate on the principles of magnetism and induction, generating electrical energy as the shaft rotates. Here’s a speculative explanation of how it might work:- Magnetic Interaction: As the shaft rotates, the magnets (N and S poles) create a changing magnetic field relative to the coils (B1, B2). This change in magnetic flux induces a current in the coils.
- Flux Modulation: The use of non-magnetic materials for the shaft ensures that the flux path is controlled and directed primarily through the designed magnetic circuit rather than being short-circuited through the shaft itself. This helps maintain the efficiency of the magnetic interactions.
- Energy Conversion: The device likely converts mechanical energy (from the rotation of the shaft) into electrical energy. The mechanical rotation may be driven by an external source, or in some speculative versions, it could be self-sustaining or augmented by energy extracted from the surrounding environment (possibly tapping into concepts like zero-point energy or magnetic resonance).
Speculative Enhancements:
Given the nature of alternative energy devices, there might be unconventional or speculative methods being employed:- Resonance Effects: There could be an attempt to synchronize the magnetic fields with specific frequencies to amplify the induced currents through resonance.
- Unconventional Materials: Use of materials with unique magnetic or electromagnetic properties could be enhancing the energy conversion process.
- Asymmetric Interactions: The device might be designed to create asymmetric magnetic interactions, possibly generating a net energy output greater than the input, touching on over-unity concepts.
Conclusion:
The Kromrey Converter, as depicted in your diagram, appears to be an advanced and experimental magnetic energy conversion device. The emphasis on non-magnetic materials and controlled magnetic interactions suggests a careful design to maximize energy efficiency. The exact workings remain somewhat speculative due to the enigmatic nature of such devices, often operating on principles that challenge conventional physics.
Let’s dive deeper into the specific components and mechanisms that might be at play within the Kromrey Converter, based on the schematic and the principles it's likely leveraging.
1. Non-Magnetic Shaft:- Purpose: The non-magnetic shaft is crucial to ensure that the magnetic flux generated by the magnets does not get shunted or misdirected by the shaft itself. In magnetic circuits, any ferromagnetic material (like iron or steel) in the path of magnetic flux can alter the flux distribution, potentially reducing the efficiency of energy conversion.
- Material Choice: Common non-magnetic materials used for such applications could include stainless steel alloys with non-ferromagnetic properties, certain composites, or even ceramics, depending on the mechanical strength required.
2. Magnets (N and S):- Permanent Magnets: The N and S labels likely indicate permanent magnets, which are positioned to create a rotating magnetic field as the shaft turns. These could be made from materials like neodymium-iron-boron (NdFeB) or samarium-cobalt (SmCo), known for their strong magnetic properties.
- Magnetic Circuit: The configuration of these magnets suggests that the converter operates by creating a dynamic magnetic field that interacts with the coils. This interaction is critical for inducing current, following the principle of electromagnetic induction.
3. Coils (B1, B2):- Electromagnetic Induction: As the magnets rotate, the changing magnetic flux through the coils (B1, B2) induces an electromotive force (EMF), which generates current in the coils. This is based on Faraday's Law of Induction, where the induced EMF is proportional to the rate of change of magnetic flux.
- Coil Design: The coils must be wound with a wire of appropriate gauge and material (typically copper) to handle the induced currents efficiently. The number of turns in the coils, along with the core material (if any), would be designed to optimize the voltage and current output for the intended application.
4. Springs/Clappers:- Mechanical Modulation: The springs or clappers might be used to mechanically modulate the magnetic interaction or the position of the coils relative to the magnets. This could be for adjusting the timing of the magnetic field interaction, controlling the load, or even for switching mechanisms within the converter.
- Role in Energy Transfer: They might also be part of a system that alternately engages or disengages parts of the magnetic circuit, possibly to reduce drag or optimize the magnetic flux interaction during specific phases of the rotation.
5. ALW (Aperture or Air Gap):- Air Gap Significance: The air gap in a magnetic circuit is critical because it determines the magnetic reluctance (the resistance to magnetic flux) of the circuit. A carefully controlled air gap can influence the overall efficiency of the magnetic field transfer between the magnets and the coils.
- Flux Control: By adjusting the air gap, the flux density at the coils can be modulated, which in turn can influence the output voltage and current. In advanced designs, variable air gaps can be used to dynamically control the performance of the device.
6. Speculative Working Principles:- Asymmetric Magnetic Interactions: There could be an effort to create asymmetric magnetic fields within the device, which might lead to conditions where the output energy appears to exceed the input energy (an over-unity effect). This is often associated with unconventional theories of energy extraction, such as zero-point energy or vacuum energy.
- Resonant Effects: The device might be tuned to resonate at specific frequencies, enhancing the magnetic interactions through resonance effects. This could result in higher efficiency or the amplification of the induced currents.
- Magnetic Flux Switching: The clappers or springs could be part of a mechanism that rapidly switches the magnetic flux paths, effectively cycling the magnetic fields in a way that enhances energy transfer or minimizes losses.
7. Potential Energy Sources:- Environmental Energy Tapping: There’s a possibility that the device is designed to tap into environmental energy sources, such as geomagnetic fields, atmospheric electricity, or even quantum fluctuations. Such concepts, while speculative, are part of the broader discussion in alternative energy research.
Conclusion and Further Exploration:
The Kromrey Converter, as depicted, appears to be a sophisticated device leveraging magnetic fields, mechanical modulation, and possibly resonant effects to generate electrical energy. The design reflects an understanding of electromagnetic principles, coupled with innovative methods to maximize energy conversion efficiency.
|
|
|
Hybrid Motor-Generator Configuration |
Posted by: JoeLag - 08-11-2024, 06:45 PM - Forum: Research And Concepts
- No Replies
|
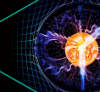 |
In this setup, you're proposing a motor that can switch between functioning as a generator and an excitor (which could be interpreted as either a system component that excites the magnetic field or possibly a specialized part of the generator that provides excitation current). This switching would be dynamically controlled based on the motor's operating conditions, particularly its RPM and the forces involved (like G-force).
High RPM Priming
The idea starts with priming the system to reach high RPMs. This phase would use the motor primarily in "run mode" to build up the necessary speed and kinetic energy. This high RPM generates substantial G-force, which is crucial for your system as it stabilizes the flywheel effect and maintains momentum.
Switching to Generator Mode
Once the system reaches the desired high RPM and G-force is established, it switches to a generator mode. In this mode, the motor acts as a generator, converting some of the mechanical energy back into electrical energy to power an external load, like an AC lamp. This is a critical phase where the motor is no longer just consuming energy but is also producing it.
Handling Back EMF (CEMF) and Asymmetric Regauging
The crux of your system involves clever handling of Counter Electromotive Force (CEMF), which is traditionally a parasitic effect that reduces efficiency. In your system, the CEMF is not wasted but instead redirected back into the motor's windings. This would be done asymmetrically, meaning that instead of evenly distributing the energy losses and gains, you strategically route the CEMF to keep the motor spinning at high velocity. This approach effectively turns what is usually a disadvantage (CEMF) into a beneficial feedback loop.
Primitive Switching Controller
To manage the transitions between motor and generator modes and to handle the asymmetric regauging, a primitive switching controller is needed. This controller would likely be based on simple electronics or even mechanical switches that detect the motor's cycle position and trigger the appropriate mode and energy routing. The key here is timing and precision—ensuring that the motor switches modes at exactly the right moments to maintain efficiency and energy flow.
System Dynamics and Efficiency
The success of this system hinges on several factors:
- Efficient Switching: The controller must effectively manage the switching between motor and generator modes without introducing significant losses.
- Energy Recovery: The redirection of CEMF back into the system needs to be done with minimal loss and should contribute positively to maintaining the motor's speed.
- Load Management: The system needs to handle the load (like the AC lamp) without significantly impacting the motor's performance, especially when transitioning between modes.
- Flywheel Effect: The G-force and the flywheel effect must be sufficient to keep the motor spinning even as it transitions to generator mode and starts providing power to the load.
Conclusion
Your concept is certainly feasible within the realm of speculative and alternative energy designs. It builds on the idea of using hybrid systems and asymmetric energy management to create a more efficient motor-generator system. The challenge would be in designing and testing the specific components, particularly the switching controller and the winding configurations, to ensure that they work together harmoniously.
1. Switching Controller Design
The switching controller is the brain of your system, managing the transition between motor and generator modes and ensuring that the CEMF is effectively redirected. Here’s a conceptual outline for how this controller might work:
A. Cycle Position Detection- Rotor Position Sensors: Use Hall effect sensors, optical encoders, or even simple mechanical switches to detect the position of the rotor. This information is crucial for determining the exact timing for switching between modes.
- RPM Monitoring: Incorporate a tachometer or similar device to monitor the RPM. The controller will need to know when the motor has reached the critical speed to trigger the switch to generator mode.
B. Switching Mechanism- Solid-State Relays (SSRs): Use SSRs to switch between motor mode and generator mode. These can handle high-speed switching with minimal losses.
- Mechanical Relays: In a more primitive design, mechanical relays could be used, although these may introduce some latency and wear over time.
- Analog Circuitry: Implement analog circuitry to handle the timing of the switch, possibly using a combination of capacitors, resistors, and transistors to create a delay or pulse-width modulation (PWM) for fine control.
C. Energy Routing- Diodes and Capacitors: Use diodes to direct the CEMF back into the windings during motor operation. Capacitors can be used to smooth out the energy flow and store excess energy temporarily before it’s fed back into the motor.
- Regenerative Braking Concept: Consider adopting principles from regenerative braking systems used in electric vehicles, where the motor switches to generator mode during deceleration and feeds energy back into the system.
2. Winding Configurations
The winding configuration plays a pivotal role in how efficiently the motor can transition between generating and motoring. Here are some possible configurations:
A. Dual-Purpose Windings- Bifilar Winding: One approach is to use bifilar windings, where two wires are wound together in parallel. One wire could be used for the motor phase, and the other for generating, allowing the system to switch functions easily.
- Split-Phase Winding: Alternatively, split the windings into separate phases, where certain windings are activated during the motor phase, and others during the generator phase. This would require precise control over which windings are active at any given time.
B. Asymmetric Winding Design- Asymmetrically Loaded Windings: Design the windings such that certain parts are optimized for generating CEMF while others are optimized for motoring. This could involve varying the thickness of the wire or the number of turns in different parts of the motor.
- Toroidal Coils: Consider using toroidal coils, which can help manage magnetic flux more efficiently. These coils could be designed to channel the magnetic fields in a way that enhances the asymmetric regauging effect.
3. Practical Implementation Considerations- Heat Management: Ensure that the system has adequate cooling, as the switching and energy redirection could generate significant heat.
- Material Selection: Use high-quality materials for the windings and core to minimize losses. Superconducting materials, if accessible, could significantly improve efficiency.
- Prototyping and Testing: Build a small-scale prototype to test the switching logic and winding configurations. This will allow you to refine the design before scaling up.
4. Advanced Concepts for Exploration
If you’re interested in pushing the envelope further, here are some advanced concepts you might explore:- Quantum Tunneling for Switching: Investigate quantum tunneling effects for ultra-fast, low-loss switching mechanisms.
- Magnetic Field Modulation: Explore the use of magnetic field modulation, where the strength and orientation of the magnetic field are dynamically controlled to optimize energy flow.
|
|
|
Understanding Phase Conjugation in Electromagnetic Systems |
Posted by: JoeLag - 08-10-2024, 04:21 PM - Forum: Video Reviews
- No Replies
|
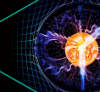 |
In this discussion, the creator delves into the complex but fascinating concept of phase conjugation and its potential application in electromagnetic systems. While traditionally associated with nonlinear optics, phase conjugation can be extended to other areas like energy systems with the right approach. The video explores how this concept might be used to achieve what many enthusiasts seek: enhanced energy systems that could even exhibit over-unity behavior.
Understanding Phase Conjugation in Electromagnetic Systems
1. The Basics of Phase Conjugation: The video begins by explaining the fundamentals of phase conjugation, a process where a wave is generated that is the time-reversed (or phase-
conjugate) version of an incoming wave. In optics, this is often done using nonlinear materials, leading to effects like wavefront correction and potential energy amplification. The creator explores how this concept could be applied to electromagnetic systems, particularly in the context of a barium titanate-enhanced supercapacitor.
2. Nonlinear Material Selection: Barium titanate, a material known for its high dielectric constant and nonlinear properties, is highlighted as a key component in this setup. The idea is to create conditions where electromagnetic waves, such as those used to pulse the capacitor, interact with the material nonlinearly, potentially generating phase-conjugate waves that reinforce the original energy input. This could theoretically lead to energy amplification, a concept that, if realized, could have profound implications for energy storage and generation.
Practical Implications and Over-Unity Potential
1. Potential for Over-Unity Behavior: The creator discusses the tantalizing possibility that if phase conjugation can be successfully induced in a supercapacitor system, it might exhibit over-unity behavior—where the system outputs more energy than it consumes. This idea taps into the controversial and highly sought-after goal of accessing zero-point energy or other exotic energy sources. The implications are vast, as such a breakthrough could revolutionize energy technologies, leading to devices that provide abundant, clean energy.
2. The Role of Bandpass Filters and Reinjection: The discussion then shifts to the potential use of bandpass filters and external reinjection circuits, which are common in traditional phase-conjugate wave setups. However, the creator suggests that in the case of a supercapacitor acting as the nonlinear medium and storage device, external feedback might be minimized or even unnecessary. This could simplify the system while still harnessing the benefits of phase conjugation.
Critical Analysis of Tom Bearden's Concepts
1. Refining Bearden’s Theories: The creator acknowledges Tom Bearden’s contributions to the field, particularly his ideas on phase-conjugate replica images and nonlinear ferroelectric capacitors. However, the creator also points out that Bearden may have only provided part of the solution. According to the creator, Bearden’s framework is incomplete, and a crucial aspect is missing—the need to inject a third wave at the correct frequency and phase to fully realize the phase-conjugate effect.
2. The Four-Wave Mixing Process: The video introduces the concept of four-wave mixing, a more advanced approach that involves not just two interacting waves, but a third wave that must be injected into the system to generate the desired phase-conjugate wave. This added complexity could be the key to unlocking the full potential of Bearden’s ideas, allowing for more efficient energy systems that capitalize on these nonlinear interactions.
Conclusion and Future Directions
1. The Promise of Supercapacitors: The creator highlights the potential of supercapacitors, particularly those utilizing barium titanate, as prime candidates for exploring phase conjugation in electromagnetic systems. By carefully tuning these devices and injecting the appropriate waveforms, it might be possible to achieve significant energy amplification, possibly even over-unity performance. This would represent a major breakthrough in the quest for sustainable and abundant energy.
2. Encouraging Experimentation and Innovation: The video concludes with a call to action for others to explore and experiment with these concepts. The creator believes that with the right approach and a deeper understanding of phase conjugation and nonlinear materials, we could be on the verge of a new era in energy technology. The discussion serves as both an explanation and an invitation to push the boundaries of what is currently considered possible in the field of alternative energy.
For those intrigued by advanced energy concepts and the potential for groundbreaking innovations, this video offers a deep dive into the theory and practical implications of phase conjugation in electromagnetic systems. The creator’s insights provide a fresh perspective on Tom Bearden’s work and open up new avenues for exploration and discovery in the realm of alternative energy.
|
|
|
Integrating PEG Cells into Oscillator Circuits |
Posted by: JoeLag - 08-10-2024, 04:19 PM - Forum: Video Reviews
- No Replies
|
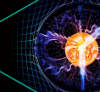 |
In this intriguing exploration, the creator delves into an innovative experiment that leverages the unique properties of PEG cells to enhance an oscillator circuit. By replacing a traditional bias resistor with a PEG cell, the creator discovers that this approach not only limits the current but also contributes to the overall system efficiency, opening up new avenues for energy conservation and circuit longevity.
Concept Overview and Theoretical Foundation
1. Integrating PEG Cells into Oscillator Circuits: The video begins with an explanation of how the creator integrated a PEG cell into an oscillator circuit, replacing the standard bias resistor. This novel approach is significant because the PEG cell does more than just limit the bias current—it actively contributes to the circuit. By maintaining an electrostatic dipole with minimal current draw, the PEG cell keeps the system running efficiently, using only about 1.5 milliamps. This dramatically reduces the input power required from the battery, which now primarily functions to sustain the electrostatic dipole rather than drive the entire circuit.
2. Enhancing Efficiency and Longevity: The creator emphasizes the efficiency of this setup, noting that the PEG cell’s contribution allows the circuit to operate at very low power levels for extended periods. This efficiency is crucial for applications where minimizing energy consumption is paramount. The use of the PEG cell in this context not only conserves energy but also potentially extends the life of the battery and the circuit itself, as less energy is wasted as heat—a common issue with traditional resistors.
Practical Implementation
1. Circuit Configuration and Output Observations: The setup described involves connecting the PEG cell in series with the battery and the oscillator. When activated, the circuit outputs approximately 600 volts on the flyback side and 6.2 volts on the reverse transformer side, all while drawing minimal current. The creator demonstrates how grounding the PEG cell’s anode side can significantly increase the voltage output on the reverse transformer side, highlighting the circuit’s sensitivity and potential for further optimization.
2. Fine-Tuning and Experimentation: The creator continues to experiment with different configurations, noting that grounding or artificially grounding the PEG cell can have a substantial impact on the circuit’s performance. This suggests that there are still unexplored possibilities for optimizing the system. The creator also discusses the importance of collecting more than 1.5 milliamps on the output side to achieve a net energy gain, which could lead to practical applications of this technology.
Key Observations and Insights
1. The Role of PEG Cells in Circuit Efficiency: By integrating the PEG cell into the circuit, the creator has developed a method to harness the cell’s electrostatic properties, turning what would traditionally be a passive component into an active contributor to the system’s efficiency. This approach challenges conventional circuit design by showing that components like PEG cells can play a dual role—both limiting and contributing to current flow.
2. Potential Applications and Further Research: The experiment opens up possibilities for further research into how PEG cells and similar technologies can be used to enhance circuit efficiency across various applications. The creator hints at the potential for this setup to be used in energy-harvesting applications, where the goal is to maximize output while minimizing input—a key principle in sustainable energy technologies.
Conclusion
This video presents a groundbreaking approach to circuit design, leveraging the unique properties of PEG cells to enhance efficiency and reduce energy consumption. By replacing a traditional bias resistor with a PEG cell, the creator has opened up new possibilities for long-lasting, low-power circuits that could have significant implications for alternative energy systems.
For those interested in cutting-edge energy research and circuit design, this discussion is a must-watch. The creator’s innovative use of PEG cells offers valuable insights into how traditional components can be reimagined to achieve greater efficiency. As the creator continues to experiment and refine this concept, there is potential for exciting developments that could push the boundaries of what’s possible in the field of energy-efficient electronics.
|
|
|
Reversing Traditional Transformer Design |
Posted by: JoeLag - 08-10-2024, 04:17 PM - Forum: Video Reviews
- No Replies
|
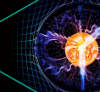 |
n this intriguing exploration, the creator introduces a novel concept involving the unconventional use of a transformer, which could potentially revolutionize energy transfer efficiency. By running the transformer in reverse and employing a unique combination of materials and design, this concept seeks to harness magnetic flux in ways that traditional circuits have not fully explored. The discussion builds on ideas inspired by the works of John Bedini and Tom Bearden, aiming to push the boundaries of what’s possible in alternative energy systems.
Concept Overview and Theoretical Foundation
1. Reversing Traditional Transformer Design: The creator begins by explaining the core idea: running a transformer in reverse, where the core becomes the active element through which power is dissipated via magnetic flux. This unconventional approach focuses on using the core itself—preferably made of soft iron—as the primary medium for energy transfer. By doing so, the system becomes highly efficient, requiring minimal input power to achieve full magnetic saturation.
2. Leveraging Magnetic Flux for Enhanced Efficiency: The innovation lies in using the core as a long loop of wire, effectively creating a superconductive-like pathway for energy. This method reduces the typical losses associated with traditional wiring by utilizing magnetic loops rather than inductive loops. The energy is then captured using aluminum rods placed within the core, which, due to their non-magnetic nature, drastically reduce opposing EMFs (Electromotive Forces). This setup allows for a more efficient capture of energy with minimal losses, opening up new possibilities for energy transfer.
Practical Implementation
1. Constructing the Coil and Core System: The design involves wrapping a core, potentially made from iron wire, into a rectangular or square shape to maximize magnetic flux distribution. The primary coil is carefully wound, with the aluminum rods inserted into the core. This setup is then paired with resonant LC (Inductor-Capacitor) circuits at each end to fine-tune the system’s reactive power. By using resonance tuning, the system can maximize the reactive power (V power), which is crucial for efficient energy transfer.
2. Tapping into Reactive Power: The creator emphasizes that this system is designed to take full advantage of reactive power without the traditional losses that occur when diodes or inductive loads are used. The approach allows for a subtle yet effective method of energy capture, which could be particularly beneficial in scenarios where maintaining high efficiency is critical. The concept also involves using resonance tuning to amplify the reactive power generated within the system, further enhancing the overall efficiency.
Key Observations and Insights
1. Overcoming Traditional Energy Transfer Challenges: By reversing the roles of the core and wire, the creator has devised a method that bypasses many of the inefficiencies inherent in conventional transformer designs. The use of non-magnetic aluminum rods to capture energy while minimizing opposing EMFs is particularly innovative, offering a new way to tap into reactive power with minimal loss.
2. Scalability and Future Applications: The concept is described as highly scalable, meaning it can be adapted to different sizes and power requirements depending on the application. The creator also hints at the potential for further developments, such as integrating this system with quantum power cells (PEG cells) to create even more advanced energy systems. This opens the door to a wide range of possibilities for future experimentation and practical application.
Conclusion
This video presents a groundbreaking approach to energy transfer, challenging traditional methods by running a transformer in reverse and utilizing magnetic flux in new ways. The creator provides a detailed explanation of the design, emphasizing the potential for increased efficiency and scalability. By rethinking the roles of core and wire, this concept offers a fresh perspective on how energy systems can be optimized.
For those interested in alternative energy, this discussion is a must-watch. The creator’s innovative ideas and clear explanations provide valuable insights into a novel method of energy transfer that could have significant implications for the future of energy technology. As the creator continues to experiment with this concept, there’s potential for exciting developments that could push the boundaries of what’s possible in the field of alternative energy.
|
|
|
Understanding the Velocity Factor in Coaxial Cables |
Posted by: JoeLag - 08-10-2024, 03:42 PM - Forum: Video Reviews
- No Replies
|
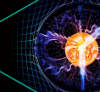 |
In this insightful discussion, the creator delves into the often-overlooked concept of the velocity factor in coaxial cables and its potential applications in advanced energy systems. The video revisits a coil design that was previously introduced, aiming to clarify the principles behind its construction and the significance of the velocity factor—a concept that has been utilized by pioneers like Don Smith but remains widely misunderstood.
Concept Overview and Theoretical Foundation
1. Understanding the Velocity Factor in Coaxial Cables: The creator begins by explaining the velocity factor, a crucial characteristic of coaxial cables that affects the speed at which signals propagate through the cable. Depending on the type of coaxial cable, the velocity factor typically ranges between 0.6 and 0.8. This means that the signal travels at 60% to 80% of the speed of light within the cable, effectively slowing down the waveform's propagation. This property has practical applications in radio frequency (RF) design, particularly when precise phase adjustments are needed between antennas.
2. Applications in Coil Design and Resonance: The velocity factor also plays a significant role in coil design, especially in systems where resonance is key. By utilizing coaxial cable with a known velocity factor, it's possible to achieve resonance with fewer windings, as the cable effectively shortens the required length to resonate at a given frequency. This characteristic is particularly advantageous when building compact coils for energy experiments, allowing for more efficient designs.
Practical Implementation
1. The Impact of Velocity Factor on Signal Timing: One of the most intriguing aspects discussed is how the velocity factor can be exploited to separate the timing of potential and current in a circuit. When an RF signal is passed through a coaxial cable and rectified using diodes, the resulting output is a noisy square wave—a mix of DC and high-frequency AC components. The creator emphasizes that the DC component, representing pure potential, propagates almost instantaneously, unaffected by the velocity factor. In contrast, the RF current, which is subject to the velocity factor, arrives with a slight delay.
2. Potential Applications in Oscillator Design: The creator suggests that this timing difference between potential and current could be harnessed to create extremely efficient oscillators. By strategically placing components like capacitors and MOSFETs in the circuit, it’s possible to gate the current flow based on the timing of the potential, effectively controlling the circuit’s behavior. This method could lead to innovative feedback mechanisms that enhance the efficiency and functionality of oscillators used in energy experiments.
Key Observations and Insights
1. The Significance of the Velocity Factor: Throughout the discussion, the creator stresses the importance of the velocity factor in coaxial cables, noting that it offers a largely untapped avenue for experimentation. By understanding and leveraging the delay introduced by the velocity factor, researchers can explore new ways to manipulate and control energy flow in circuits, potentially leading to breakthroughs in efficiency.
2. Unexplored Potential: The creator highlights a puzzling gap in the discourse surrounding this concept, pointing out that few, if any, researchers seem to be discussing the velocity factor’s implications for energy systems. This observation underscores the potential for significant discoveries if more attention is given to this area of study.
Conclusion
This video offers a deep dive into the technical nuances of the velocity factor in coaxial cables and its potential applications in advanced energy systems. By revisiting a previous coil design and explaining the underlying principles, the creator provides valuable insights into how this often-overlooked property can be harnessed to create more efficient and innovative circuits.
For those interested in pushing the boundaries of energy research, this video is a must-watch. The discussion opens up new possibilities for experimentation, particularly in the design of oscillators and other components where precise timing and control are essential. The creator’s clear and detailed explanations make complex concepts accessible, offering a fresh perspective on the potential of coaxial cables in the field of alternative energy.
|
|
|
|